Syndesmotic Ankle Sprains
A syndesmotic ankle sprain, also known as a high ankle sprain, is a specific type of injury that affects the ligaments connecting the tibia and fibula, the two bones in the lower leg.
Definition:
A syndesmotic, or ‘high’ ankle sprain is one that involves the ligaments binding the distal tibia and fibula at the Distal Tibiofibular Syndesmosis.
Injuries can occur with any ankle motion, but the most common motions are extreme external rotation or dorsiflexion of the Talus.
The dome of the Talus is wider in the anterior than in the posterior, and these movements force apart the medial and lateral aspects of the mortise, respectively the tibial and fibular malleoli.
The sufficient distraction of the distal fibula from the tibia can cause strain or rupture of one or more of the following ligaments: the anterior inferior tibiofibular ligament, superficial posterior inferior tibiofibular ligament, transverse tibiofibular ligament, interosseous membrane, interosseous ligament, and inferior transverse ligament.
Rupture injuries also commonly present with concomitant fractures of either malleolus (lateral being more common) or proximal fibular spiral fracture is known as a Maissonneuve fracture.
Anatomy related to Syndesmotic Ankle Sprains
To understand the tibiofibular syndesmosis, a thorough knowledge of the surrounding anatomic structures is needed.
A detailed and comprehensive understanding of the superficial and deep structures of the talocrural and subtalar joints, in addition to the tibiofibular articulations, is needed to fully comprehend the joint mechanics and injury mechanisms involved.
Talocrural and Subtalar Joints:
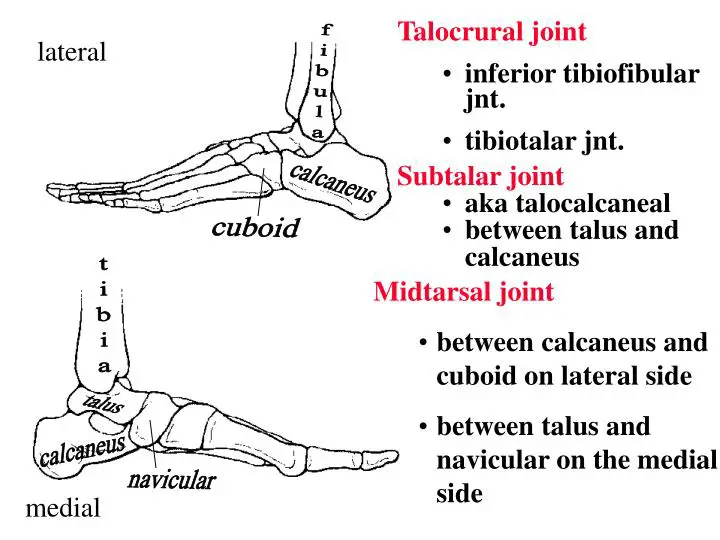
The talocrural, or ankle joint, is a uniaxial, modified-hinge joint formed by the talus, the medial malleolus of the tibia, and the lateral malleolus of the fibula.
Specifically, the concave distal articular facet of the tibia articulates with the convex superior articular surface of the talus, or trochlea.
The medial malleolus articulates with the medial aspect of the trochlea, whereas the lateral malleolus articulates with the lateral aspect of the trochlea.
The stability of the ankle mortise is enhanced because the dome-shaped body of the talus fits snugly into the slightly concave tibial undersurface
Just inferior to the talocrural joint is the subtalar joint.
This joint lies beneath the talus, where the posterior calcaneal facet on the talus articulates with the posterior facet on the superior aspect of the calcaneus.
The subtalar joint is a gliding joint, with the 2 bones held together by an articular capsule and by anterior, posterior, lateral, medial, and interosseus talocalcaneal ligaments. Subtalar inversion and eversion occur at this articulatio.
The relation of the tibia, fibula, and talus is maintained by an articular capsule and 3 groups of ligaments (medial, lateral, and syndesmosis).
The articular capsule surrounds the joint and is attached to the borders of the articular surfaces of the malleoli proximally and to the distal articular surface of the talus distally.
The anterior aspect of the capsule is broad, thin, and membranous, whereas the posterior component of the capsule is very thin and consists mostly of transverse fibers.
The lateral aspect of the capsule is slightly thickened.
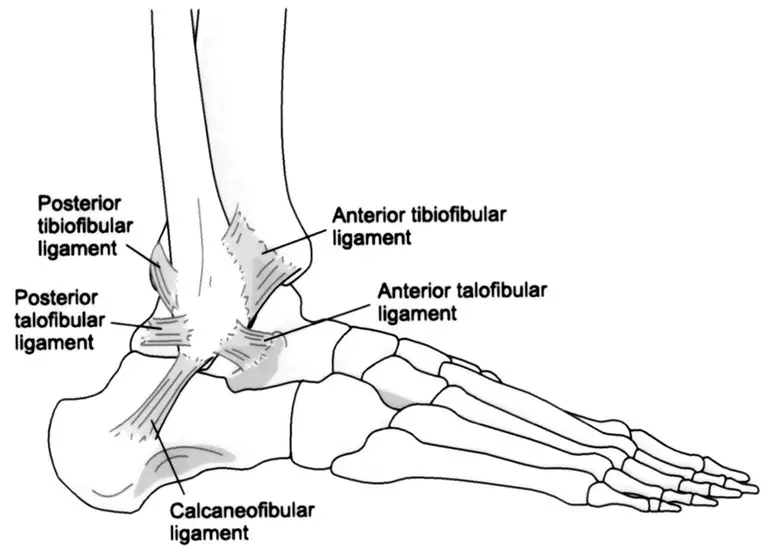
The deltoid ligament is a strong, flat, and triangularly shaped ligament on the medial aspect of the ankle.
This ligament consists of 4 bands: the anterior tibiotalar, the posterior tibiotalar, the tibiocalcaneal, and the tibionavicular. The deltoid ligament is considered the strongest of the ankle ligaments and, especially during plantar flexion, functions to prevent excessive eversion at the subtalar joint.
The deltoid, particularly its anterior portions, also resists talar external rotation.
The lateral malleolus extends further distally than does the medial malleolus and, as a result, provides a bony limitation against excessive eversion.
Three lateral ligaments aid in preventing excessive inversion at the subtalar joint.
The anterior talofibular ligament, the posterior talofibular ligament, and the calcaneofibular ligament make up the lateral collateral ligaments of the ankle.
The anterior talofibular ligament limits anterior displacement and medial shifting of the talus and posterior displacement and lateral rotation of the tibia and fibula, respectively, primarily in plantar flexion.
This ligament also helps to prevent lateral talar tilt. The posterior talofibular ligament braces the talus posteriorly and helps to limit talar external rotation (or internal rotation of the tibia and fibula).
The calcaneofibular ligament functions to prevent lateral talar tilt, principally when the ankle is in a neutral amount of plantar flexion and dorsiflexion.
The bony and ligamentous arrangement of the talocrural joint provides it with considerably more stability than other diarthrodial joints, such as the knee or shoulder.
Depending on the position and the loads placed on the joint, the bones and ligaments alternate as primary and secondary stabilizers.
Weight-bearing and axial loading have been reported to increase talocrural bony stability.
When dorsiflexed, the ankle is thought to be in the most stable position, sometimes termed close-packed, since this is the position of the most bony contact.
In this position, most of the mortise is occupied by the talus, and contact is maximal between the involved articulating surfaces.
Tibiofibular Syndesmosis:
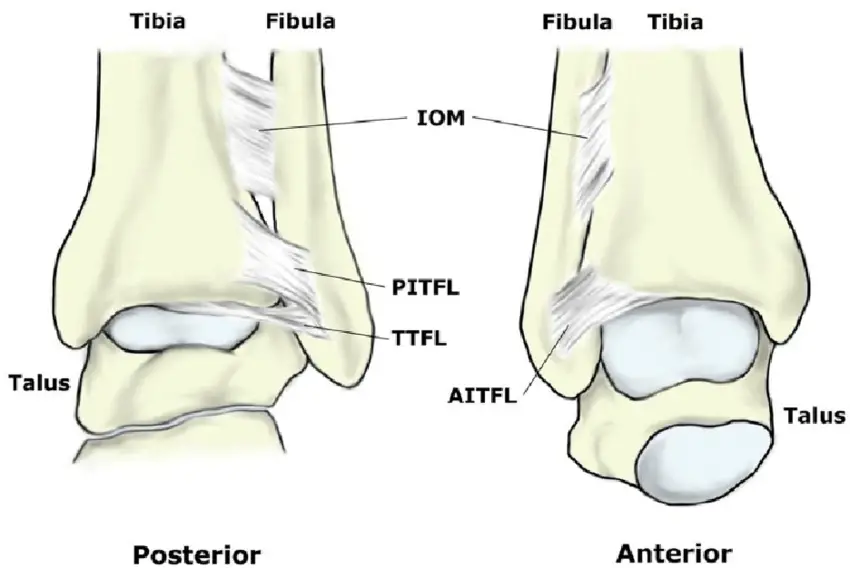
A third articulation in the region of the ankle and lower leg is between the tibia and fibula.
This articulation of the fibula with the tibia can be subdivided further into 3 regions: the superior or proximal tibiofibular joint, the interosseous membrane, and the inferior or distal tibiofibular joint.
The superior tibiofibular joint is a syndesmotic joint that is held in place by the anterior superior tibiofibular and posterior superior tibiofibular ligaments.
This articulation helps to maintain proximal integrity between the tibia and fibula.
The interosseous membrane holds the fibula and tibia together.
This membrane also stabilizes any posterolateral bowing of the fibula that may occur with weight bearing.
This membrane is a thick osseofascial structure extending from the tibial periosteum to the fibula, nearly the entire length between the 2 bones.
Anteriorly, the fibers are parallel and run obliquely downward from the tibial interosseous ridge at approximately a 15° to 20° angle. Posteriorly,
the fibers are closer to vertical as they run from the tibia to the fibula.
Skraba and Greenwald21 investigated the role of the interosseous membrane in stress transmission to the fibula.
The loads experienced in normal gait were reproduced using 3 cadaver legs fitted with strain gauges.
The interosseous membrane was found to play an important role in the transfer of forces to the fibula.
After the membrane was incised, strains recorded in the fibula decreased to approximately 0.
These results suggest that an intact interosseous membrane keeps the fibula active during weight bearing and that this structure plays an active role in normal tibiofibular function.
Thomas et al investigated the roles of the fibula and interosseous membrane on compressive load sharing in the lower extremity.
Twelve fresh-frozen cadaver lower extremities were evaluated intact, after interosseous membrane sectioning, and after partial fibula excision.
The specimens were also tested in different ankle and subtalar positions.
Thomas et al,19 in contrast to Skraba and Greenwald,21 found that fibular strain was not reduced to 0 after sectioning of the interosseous membrane, indicating that loads are transmitted at the proximal and distal tibiofibular articulations.
Inferior Tibiofibular Joint:
The inferior tibiofibular joint is defined as a syndesmotic articulation between the convex surface of the distal fibula and the concave distal tibia.
The distal fibula is firmly attached at the fibular notch of the tibia by several syndesmotic ligaments.
The stability of this articulation is integral in allowing for the proper functioning of the ankle and lower extremities.
The ligaments that stabilize this joint are the anterior inferior tibiofibular ligament, the posterior inferior tibiofibular ligament, and the interosseous ligament.
The most distal and inferior aspect of the interosseous membrane also helps to stabilize this joint.
The anterior inferior tibiofibular ligament is a flat, strong ligament. It originates from the longitudinal tubercle on the anterior aspect of the lateral malleolus, and the fibers course superiorly and medially, attaching to the anterolateral tubercle of the tibia.
The fibers of this ligament increase in length from proximal to distal, with the most distal fibers being the longest.
In addition to holding the fibula tight to the tibia, this ligament prevents excessive fibular movement and external talar rotation.
The posterior inferior tibiofibular ligament has superficial and deep components.
The superficial fibers originate widely on the posterior tubercle of the tibia and run obliquely, distally, and laterally to the posterior lateral malleolus.
This ligament works with the anterior inferior tibiofibular ligament to hold the fibula close in the fibular groove of the tibia.
The deep component of the posterior ligament is the transverse tibiofibular ligament.
Some anatomists consider this ligament to be independent of the posterior inferior tibiofibular ligament.
The transverse ligament is a thick, strong structure with twisting fibers.
It passes from the posterior tibial margin to the osteochondral junction on the posterior and medial margins of the distal fibula.
The location of the transverse ligament below the posterior tibial margin helps it prevent posterior talar translation.
The ligament creates a posterior labrum, which deepens the articular surface of the distal tibia.
It also fills in the posteromedial aspect of the lateral malleolus, deepening the mortise and increasing joint stability.
The remaining ligament of the distal tibiofibular syndesmosis is the interosseous ligament.
Originating at the anteroinferior triangular segment of the medial aspect of the distal fibular shaft, this ligament then courses to insert on the lateral surface of the distal tibia.
The interosseous ligament is a thickening of the distal aspect of the interosseous membrane and is thought to act as a “spring,” allowing for slight separation between the medial and lateral malleolus during dorsiflexion at the talocrural joint.
Ogilvie-Harris et all studied the relative importance of each of the syndesmotic ligaments in the distal tibiofibular articulation.
They tested 8 fresh-frozen cadaver specimens on a hydraulic system to evaluate the percentage of contribution of each ligament during 2 mm of lateral fibular displacement.
The anterior inferior tibiofibular ligament provided 35%; the transverse (deep posterior) ligament, 33%; the interosseous ligament, 22%; and the superficial posterior inferior ligament, 9%.
Thus, 3 major ligamentous components provide stability to the syndesmosis, accounting for more than 90% of the total resistance to lateral fibular displacement.
Injury to one or more ligaments results in weakening, abnormal joint motion, and instability.
Biomechanics:
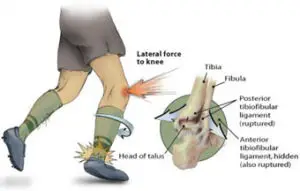
The primary motions of the ankle joint occur within the sagittal plane: dorsiflexion (ankle flexion) and plantar flexion (ankle extension).
Dorsiflexion can be described as a movement of the top of the ankle and foot toward the anterior aspect of the tibia. Plantar flexion is a movement of the ankle and foot away from the tibia.
The normal ankle allows approximately 15° to 20° of active dorsiflexion and between 45° and 55° of active plantar flexion.
10 Sarrafian16 reported about 24° of sagittal plane motion at the ankle during the stance phase of gait.
Maximal dorsiflexion is approximately 10° during the stance phase of normal running and 14° for plantar flexion.
As for most joints with passive ranges of motion greater than active ranges, the full weight-bearing ankle passively allows up to 40° of dorsiflexion.10
The articular surfaces of the talus and malleoli remain in contact as the ankle moves from dorsiflexion to plantar flexion.
The superior talar surface is wider anteriorly than posteriorly, with an average difference of 4.2 mm.
During dorsiflexion, the wider anterior portion of the talus “wedges” between the medial and lateral malleoli, and much of the mortise becomes occupied.
This position is considered the safest for the ankle due to the increased joint stability that results from this close packing of the bones and increased contact of the articular surfaces.
The wider anterior aspect of the talus moves out of the mortise during plantar flexion (loose packing), thus decreasing the ankle’s bony stability.
Despite some decreased bony stability in full plantar flexion, the posterior two-thirds of the talar dome remains in the mortise as the result of talar rotation.
Close13 documented 5° to 6° of talar external rotation during both active and passive ankle dorsiflexion.
During plantar flexion, the talus internally rotates as a result of its conical and wedged shape.
Sarrafian16 reported that, in addition to rotating internally, the talus also supinates slightly during plantar flexion.
This, in turn, causes posterolateral wedging of the talar trochlea. As plantar flexion increases, wedging between the posterolateral trochlea and lateral malleolus increases correspondingly.
During dorsiflexion, therefore, the talus must pronate, a fact that may be important in the many tibiofibular ligament injuries occurring from dorsiflexion and external rotation.
Lundberg et al,31 using roentgen stereophotogrammetry, evaluated talar motion during weight-bearing tibial rotation.
They reported triaxial talar movement when the leg (ie, tibia) was either externally or internally rotated.
Tibial external rotation of 10° caused the talus to dorsiflex 4.3° ± 3.5°, supinate 1.5° ± 1.6°, and laterally rotate 0.7° ± 2.5°.
Internal tibial rotation of 20° resulted in talar lateral rotation equal to 5.0° ± 2.0°, pronation equal to 0.7° ± 0.5°, and plantar flexion of 0.1° ± 1.9°.
These results coincide with Close’s13 earlier findings related to talar horizontal rotation.
Inversion, eversion, supination, and pronation primarily occur at the subtalar joint.
Inversion is the inward turning of the sole of the foot, whereas eversion is the outward turning. Supination is a combination of calcaneal inversion, foot
adduction, and plantar flexion, whereas pronation is calcaneal eversion, foot abduction, and dorsiflexion.
The normal ranges of motion for subtalar inversion are approximately 20° to 30°, whereas ranges for eversion are between 5° and 15°.
During ankle plantar flexion and dorsiflexion, some movement normally occurs at the distal tibiofibular syndesmosis.
When the foot is moved from a plantar-flexed position to a dorsiflexed position, the joint permits approximately 1 to 2 mm of widening at the mortise.
Movement of the fibula occurs at and affects the tibiofibular syndesmosis. While in the fibular groove of the tibia, the fibula rotates around its vertical axis when the ankle is plantar flexed and dorsiflexed.
Lateral fibular rotation is approximately 3° to 5° with dorsiflexion, and medial rotation is 3° to 5° with plantar flexion.
Mechanisms of ankle sprains :
Injury to the lateral collateral ligaments usually occurs when the athlete’s center of gravity is shifted over the lateral border of the weight-bearing leg, causing the ankle to roll inward at a high velocity.
The ATFL is the weakest of the lateral collateral ligaments and therefore the first to be injured. Injury to the CFL may also occur in more severe sprains.
Mechanisms of injury may include landing awkwardly on an opponent’s foot, catching the outer aspect of the foot on the ground terrain, or a slide tackle that contacts the inside of the opponent’s weight-bearing leg.
A study using cadaver foot and ankle models revealed tearing of the lateral collateral ligaments when the talus inverts approximately 30° to 45° within the ankle mortise.
Other structures that may be injured during a lateral ankle sprain may include the peroneal tendons, lateral joint capsule, and the proprioceptive nerve endings found within these soft tissue structures.
Literature indicates that injury to joint mechanoreceptors may occur during an ankle sprain, resulting in proprioceptive deficits and poor ankle/foot biomechanics.
Reduced mechanical strength of the ligaments and proprioceptive deficits lead to joint laxity and impaired dynamic support of the surrounding musculature.
Syndesmotic “high ankle” sprains constitute approximately 10% of all ankle sprains.
These sprains are more prevalent in high-energy contact sports or in sports that involve wearing a stiff boot or skate, such as hockey or skiing.
Examples of injury may include a skier who catches the inside edge of the uphill ski while attempting to avoid a gate or a football player who pivots suddenly to the inside with the outside foot fixed on the playing surface.
Both of these mechanisms of injury may result in an excessive external rotation force on the fibula with respect to the tibia that may lead to disruption of the syndesmotic ligaments, in particular the AITFL.
The severity of injury usually depends on the magnitude of the force and how long it was applied to the ankle mortise.
The rehabilitation period for syndesmotic injuries tends to be longer than lateral ankle injuries. A recent study indicated that a syndesmotic sprain may require a treatment period almost twice as long as a grade 3 lateral ankle sprain.
Causes
Syndesmotic ankle sprains commonly occur in athletes participating in American football and downhill skiing.
Football injuries are usually a result of forced external rotation of the foot while the athlete is prone, as in at the bottom of the pile.
The injuries can also result from a blow to the lateral knee while the foot is planted and dorsiflexed, resulting in an eversion or external rotation moment at the talocrural joint.
In downhill ski racing, the boot does not allow dorsi- or plantar-flexion movement, which can result in excessive allowance of talocrural external rotation and injury to the anterior or posterior tibiofibular ligament, as well as the interosseous membrane.
Research has documented that syndesmosis injuries account for 1-11% of all injuries. Incidence among professional American football players is reported to be much higher, up to 29% as documented by Boytim et al.
Clinical Presentation:
Observationally the Syndesmotic will show significantly less swelling than a lateral ankle sprain, as well as demonstrate a loss of full plantar flexion and an inability to bear weight.
Ecchymosis may appear several days post-injury due to the injury of the intereosseuos membrane.
Difficulty or inability to toe walk are often noted. History includes chronic pain, prolonged recovery, recurrent sprains, and the formation of heterotopic ossification within the interosseous membrane.
The most common MOI is when the foot is in external rotation with excessive dorsiflexion.
Risk factors:
High ankle sprains are much less common than the typical lateral ankle sprain.
Using clinical diagnostic criteria (ie, not radiologic), the largest observational studies report that high ankle sprains comprise approximately 6 percent of acute ankle sprains without fracture.
The incidence in the United States is 2.09 injuries per 100,000 person-years.
The incidence in United States collegiate American football players is about 2.5 per 10,000 athletic exposures.
In the general population presenting to an emergency department, 5.7 percent of acute ankle sprains without fracture are high ankle sprains.
At the United States Military Academy, 6.7 percent of acute ankle injuries involve syndesmosis, with an incidence rate of 4.8 per 1000 person-years.
A smaller study at the Academy found that 16 percent of acute ankle sprains involve syndesmosis.
The incidence reported in other subpopulations varies widely.
High ankle sprains comprised 4 percent of all acute ankle sprains among professional English soccer players, 24.6 percent among American college football players, and 73 percent among professional American hockey players.
Other studies confirm the relatively high rates among American football players and participants in other contact sports, especially wrestling and ice hockey.
The high, rigid ankle support of a hockey skate, which transfers forces proximally along the leg, likely plays a role in the higher rate of syndesmotic injury among hockey players, although the actual incidence is unlikely to be as high as that reported in the study cited above.
Examination findings of a lateral ankle sprain may include the following:
• Swelling observed distal to the lateral malleolus of the ankle that may extend to the foot if the lateral capsule is torn.
• Tenderness palpated over the ATFL and, in more severe cases, the CFL.
• The anterior drawer and the talar tilt test may reveal joint laxity due to tearing of the ATFL and/or the CFL ligament.
• Stress radiographs may reveal excessive anterior translation of the talus or inversion of the talus.
Lateral ankle sprains are based on a grade 1 to 3 classification. A grade 1 ankle sprain usually entails microscopic tearing of the ATFL.
Symptoms may include minimal swelling and point tenderness directly over the ATFL; however, there is no instability, and the athlete can ambulate with little or no pain.
A grade 2 ankle sprain involves microscopic tearing of a larger cross-sectional portion of the ATFL.
Symptoms may include a broader region of point tenderness over the lateral aspect of the ankle, a painful limp if able to ambulate, and bruising and localized swelling due to tearing of the anterior joint capsule, ATFL, and surrounding soft tissue structures.
A grade 3 ankle sprain entails a complete rupture of the ATFL and may also involve microscopic or complete failure of the CFL.
The posterior talofibular ligament is rarely injured during inversion ankle sprains.
Symptoms may include diffuse swelling that obliterates the margins of the Achilles tendon, inability to ambulate, and tenderness on the lateral and medial aspects of the ankle joint.
Examination findings of a syndesmotic sprain may include the following:
• Edema observed proximally to the ankle mortise.
• Tenderness palpated over the AITFL that may extend up the leg. The length of tenderness measured from the distal tip of the fibula proximally up the interosseous space has been called the tenderness length.
The tenderness length has been reported to correlate with the severity of a syndesmotic sprain.
• The external rotation test, squeeze test, and stabilization test results may be positive.
• Standard or stress radiographs may reveal abnormal widening of the ankle mortise.
• The external rotation test, squeeze test, and stabilization test results may be positive.
• Standard or stress radiographs may reveal abnormal widening of the ankle mortise.
Syndesmotic sprains have been separated into 3 categories:
(1) sprain without diastasis (pathological separation) of the ankle mortise.
(2) latent diastasis or diastasis that is only apparent after stress radiographs and
(3) frank diastasis that is usually accompanied by a fracture.
Dynamic ultrasound examination may prove useful in the early diagnosis and grading of syndesmotic sprains.
In a preliminary study conducted by Mei-Dan et al,39 dynamic ultrasound examination was accurate in visualizing a tear of the AITFL and abnormal widening of the ankle mortise.
If symptoms persist after 6 weeks of therapy, a computed axial tomography scan or a magnetic resonance imaging test (MRI) may be considered.
These advanced imaging tools may aid in ruling out osteochondral lesions and tumors or in reevaluating the grade of injury.
Special Testing:
1). Dorsiflexion External Rotation Stress Test (Kleiger’s Test):
Determines rotator damage to the deltoid ligament or the distal tibiofibular syndesmosis.
Performed by having the knee flexed by 90 degrees with the ankle in a neutral position and applying an external rotational force to the affected foot and ankle.
(+) test: Pain in the anterolateral ankle. An indicator of deltoid ligament damage would be if there is a displacement of the talus away from the medial malleolus.
Interrater kappa= 0.75 (best)
2). Squeeze Test:
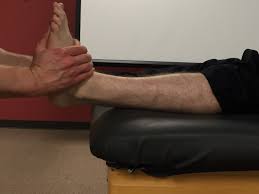
separation of the tibia and fibula.
Identifies a fibular fracture or syndesmosis sprain.
Performed by squeezing the tibia and fibula together above the injury.
(+) test: Pain will be reproduced along the fibular shaft if it’s a fibular fracture and the distal tibiofibular jt for syndesmosis sprain.
interrater= 0.5 (moderate).
3. Cotton Test:
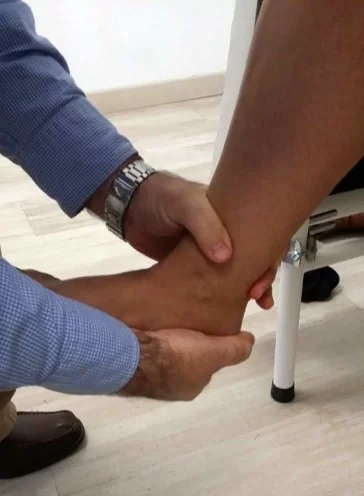
Assess for syndesmosis instability with diastasis.
Performed: steadying the distal leg with one hand while grasping the plantar heel with the opposite hand and moving the heel directly from side to side.
(+) test: Any lateral translation would indicate syndesmotic instability.
4). Fibular Traslation Tes :
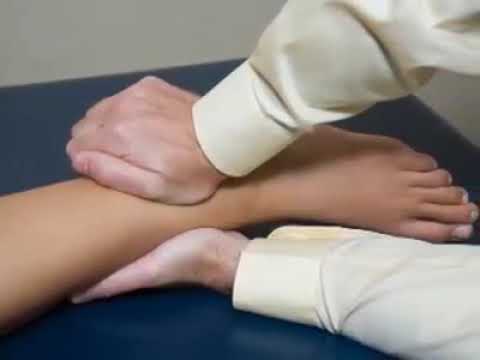
Beumer et al. (2011 ): 77% Sensitivity, 88% Specificity.
Imaging:
Radiographs:
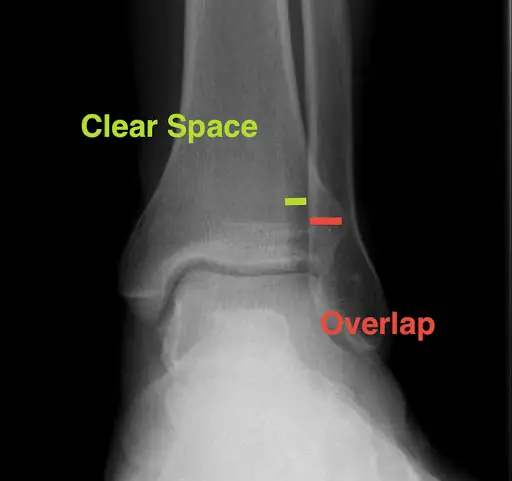
recommended views :
AP, lateral, mortise view of ankle
AP, lateral of the entire tibia
may show a fracture of the proximal fibula
optional views:
external rotation stress radiograph
gravity stress view :
will help determine the competence of deltoid ligament
contralateral ankle radiographs
may help clarify syndesmosis widening versus normal anatomic variant
findings :
decreased tibiofibular overlap
normal >6 mm on AP view
normal >1 mm on mortise view
increased medial clear space
normal less than or equal to 4 mm
increased tibiofibular clear space
normal <6 mm on both AP and mortise views
CT Scan
indications:
clinical suspicion of syndesmotic injury with normal radiographs
useful post-operatively to assess the reduction of syndesmosis after fixation
sensitivity and specificity :
more sensitive than radiographs for detecting minor degrees of syndesmotic injury
MRI:
indications :
clinical suspicion of syndesmotic injury with normal radiographs
sensitivity and specificity:
highly sensitive and specific for detecting syndesmotic injury
Management:
Stages of ligament healing:
Chronology of ligament healing has been separated into 3 distinct phases: inflammatory, reparative, and remodeling.
The following healing chronology is based on a completely ruptured ligament.
The initial response to injury would be the acute inflammatory phase, lasting 24 to 72 hours.
Damage to the blood vessels within the ruptured ligament and surrounding tissues results in a hematoma that fills the gap between the 2 retracted ligament ends.
The capillaries surrounding the injured ligament increase in permeability, resulting in swelling and the migration of plasma proteins and leukocytes (white blood cells).
The white blood cells engulf and eliminate the damaged cells and dead debris, a process termed phagocytosis.
Chemical mediators are released during phagocytosis that promote new blood vessel formation, and neovascularization.
Oxygen and nutrients are now available for the process of tissue repair.
The reparative phase of healing begins 3 to 5 days postinjury. Dead or damaged tissue is repaired or replaced with healthy cells and connective tissue.
At approximately 5 days postinjury, the gap between the 2 retracted ligament ends is filled with granulation connective tissue, consisting of macrophages, new blood vessels, and fibroblasts.
The fibroblasts produce proteoglycan and collagen, the subunits necessary for ligament repair. Collagen production intensifies; and by 10 to 14 days, disorganized collagen now connects the 2 ligament ends.
The ligament may now be able to resist low-level tensile forces.
The remodeling phase of healing begins 15 to 28 days post-injury.
The newly formed collagen fibers align themselves longitudinally, and cross-linkages form. By 3 weeks, as collagen maturation continues, the ligament may regain approximately 60% of its tensile strength.
By 3 months, the ligament may regain its preinjury strength.
The ligament healing process can be expedited by immediately instituting an aggressive nonsurgical treatment protocol that initially minimizes pain, inflammation, and swelling and incorporates early controlled motion.
The treatment approach should be individualized and under the careful observation of the treatment staff.
Studies indicate that early progressive rehabilitation has a favorable outcome in the restoration of ligament tensile strength, encourages lymphatic drainage, restores proprioception, decreases muscle atrophy, and minimizes excessive arthrofibrosis or scarring.
Treatment of grade 1 and 2 ankle sprains—for which types/severity of injuries.
The goals of therapy are to accentuate the normal healing process and protect the ligament from further injury.
The acronym PRICESMMS stands for proprioception training, rest/modified activity, ice, compression, elevation, stabilization, medications (nonsteroidal anti-inflammatory drugs), mobilization, and strength training.
These rehabilitation techniques should be applied appropriately during each phase of treatment.
Progression of the treatment plan depends on the severity of injury, the patient’s response to therapy, and the achievement of goals during each treatment phase.
Acute inflammatory phase (24-72 hours)
The initial goals of therapy are to limit inflammation, reduce pain, and protect from further injury.
• Ice therapy should be applied for 20 minutes on/1 hour off throughout the day, with a compression bandage and the leg elevated above the heart.
Cryotherapy reduces pain, edema, and secondary hypoxic damage to injured tissues.
• Nonsteroidal anti-inflammatory drugs, electric muscle stimulation, pulsed ultrasound, antiedema massage, and low-level laser therapy may help in reducing inflammation.
• Ankle pumps, 10 to 20 pumps per hour, should be conducted in a pain-free range to decrease edema and increase circulation.
• Ambulate—weight-bearing as tolerated—with axillary crutches if necessary.
A semirigid orthosis, lace-up style brace, or tape provides mechanical joint stability in the frontal plane.
• Depending on the severity of the injury, pain-free modified activity can be used to maintain cardiovascular fitness.
Examples of modified activity may include deep-water pool running, swimming with a pool buoy between the legs, or stationary cycling.
• Several studies were conducted on grade 1 and grade 2 ankle inversion injuries using manipulation of the TCJ.
Two studies showed manipulation of the talus in an anterior-to-posterior vector improved the range of motion in dorsiflexion at the ankle mortise.
Another study showed that manipulation of the joints and soft tissue improved dorsiflexion as well as reduced pain and edema.
• Active or passive soft tissue techniques such as Active Release Technique, Graston Technique, muscle energy technique, and transverse friction massage can be applied directly to the ligament and surrounding soft tissue structures to facilitate early ligament healing.
Numerous clinical studies support the use of transverse friction massage in the treatment of ligament sprains. Recently, Loghmani and Warden70 conducted a study on instrument-assisted cross-fiber massage (IACFM) and its effect on ligament healing.
Bilateral MCL injuries were induced on the knees of 51 rodents.
The IACFM has commenced with a Graston handheld tool on one of the rodent’s MCLs 1-week postinjury, at a frequency of 3 sessions per week and for a duration of a minute.
The contralateral MCL was used as the nontreated control. Histological sections were obtained 4 weeks postinjury.
The scar region of the IACFM-treated ligaments, as compared with the contralateral nontreated ligaments, appeared to have greater cellularity; and the collagen fibers appeared to be aligned more longitudinally.
This study suggests that IACFM may accelerate early tissue-level healing.
A more cautious approach to treatment may be necessary if there is suspicion of a grade 3 lateral ankle sprain.
In the opinion of one of the authors, if the initial examination reveals severe swelling, pain, and inability to bear weight, the athlete should be placed in a functional walking orthosis and instructed to ambulate with crutches in a nonweightbearing gait.
Nonsteroidal anti-inflammatory drugs, ice, compression, and elevation should be used to attempt to reduce swelling and pain. A reexamination should be conducted 3 days later.
If there is no observable improvement, an MRI study should be used to assess the severity of the injury.
If the MRI indicates a completely torn ligament, the athlete should continue to follow the above regimen for approximately 4 more days, thereby allowing the retracted ligament ends to heal appropriately before progressing to the next stage of treatment.
A brief period of nonweightbearing (1-4 days) may be necessary in the initial treatment of a syndesmotic sprain where frank diastasis of the ankle mortise is not visualized on radiographs.
Early weight-bearing may lead to undue stress on the injured syndesmotic ligaments, possibly predisposing to heterotrophic ossification in the interosseous space between the tibia and fibula.
In the case of a syndesmotic sprain that reveals diastasis of the ankle mortise greater than 6 mm, surgical fixation with screws would be necessary to stabilize the ankle mortise and allow for proper ligament healing.
An intact ankle mortise is necessary when attempting to push off the ground to propel forward or for cutting activities of most sports.
Reparative phase (3-5 days, collagen production intensifies 10-14 days)
Goals include reducing inflammation, facilitating the ligament healing process, restoring active and passive ranges of motion, minimizing loss of strength, maintaining cardiovascular fitness, and starting proprioceptive rehabilitation.
• Joint mobilization and soft tissue techniques of the TCJ and the STJ to free up joint restrictions and aid in improving range of motion.
• Passive stretch of the gastrocnemius and soleus musculature with a towel, 3 sets of 30-second holds.
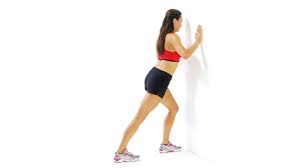
Stretching on a slant board or with a pro-stretch can be incorporated when the patient can at least partially bear weight with minimal pain.
• Isometric exercise should be conducted as soon as the patient can tolerate it to prevent muscle atrophy.
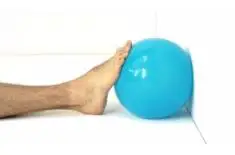
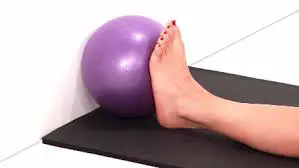
Active and passive range of motion can be conducted with minimal pain in dorsiflexion, plantar flexion, eversion, and the pain-free range of inversion.
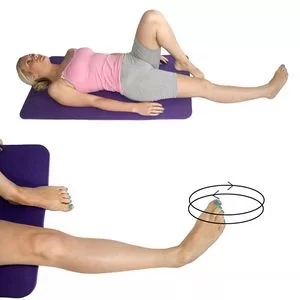
The therapist may conduct 3 sets of 10 with a 3- to 5-second hold at the passive end range.
The patient can conduct isometric exercises at home.
• Strengthen the peroneal, tibialis anterior and extensors, and the triceps surae with Thera-Band, 3 sets of 10 to 15 reps for each muscle group.
Also, strengthen the gluteus medius musculature to prevent lateral sway.
Once the patient can fully weight bear without pain, he can add resistance greater than body weight as tolerated.
This may include standing and seated calf raises.
If no weights are available, teach the patient to go up on the toes on both feet and then shift all the weight to the injured side and lower down on that leg.
• Standing proprioception training can be conducted on the floor, a wobble board, or an air-filled cushion.
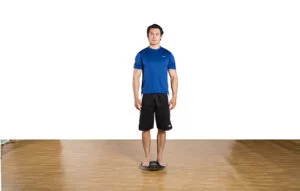
Start with single-leg standing on a flat surface, 3 times with 30-second holds; use upper extremity support from a counter if necessary.
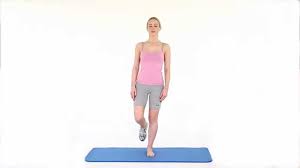
Progress to eyes closed. Progress to exercises on an air-filled cushion.
Have the patient balance on a wobble board for 30 to 60 seconds conducting various drills.
Proprioception training has been shown to reduce the occurrence and reoccurrence of ankle sprains.
• Proprioception training in the seated position with the Biomechanical Ankle Platform System (BAPS) board.
BAPS board exercises activate the peroneus longus, tibialis anterior, and gastrocnemius musculature.
73 Have the patient tap the BAPS board to the floor at 12:00, 3:00, 6:00, and 9:00 p.m. and then add 1:30, 4:30, 7:30, and 10:30 pm. Conduct 3 sets for 2 minutes each set.
The patient may also alternate counterclockwise.
When the patient can fully weight bear without pain and has demonstrated proficiency in the seated position, the BAPS exercises can be conducted standing with upper extremity support as needed.
• Shoe gear modification depends on the athlete’s foot structure and the playing surface.19
• Modified activity can be progressed appropriately.
A program to incorporate restoration of cardiovascular fitness with running may include a 5-minute warm-up with walking, followed by a 3-minute run at a very easy intensity, and then followed by a 2-minute walk; repeat run/walk repetitions for 20 to 30 minutes.
When the athlete can conduct a 20-to-30-minute run session without rest periods and there is no noticeable pain or post-swelling, the weekly mileage can be increased by 10%.
The intensity of the training runs should only progress when the athlete regains the preinjury duration.
• At the end of each treatment session, apply ice therapy and other modalities to control inflammation.
The above exercises should be conducted relatively pain-free and progressed based on the patient’s response to therapy and based on the principles of ligament healing.
After demonstrating proficiency in conducting the exercises under the supervision of a therapist, the patient can be given a home exercise routine.
If there is an excessive increase in swelling or pain, temporarily reduce the intensity or duration of the exercises followed by inflammation control.
Remodeling phase (15-28 days, 3 weeks regain ? 60% strength, 3 months regain ? 100% strength)
Goals include restoration of tensile strength, proprioception, and return to sport.
• Modified training may progress and include activities such as jumping rope, higher-intensity training runs, or sprints.
• Incorporation of multidirectional agility drills can begin at this stage. Start with controlled exercises that are on both legs; then progress to a single leg.
Progress to jumping over a height, such as a low cone. Progress to increasing in speed.
It is important to vary the speed and intensity of sport-specific exercises to continually challenge the proprioceptive system.
All progressions should be performed only as pain, comfort, and stability allow.
-Begin jumping forward and backward over a line.
-Progress to jumping laterally over a line.
-Progress to box drills: Draw a box on the floor with tape and number the boxes starting in the upper-left corner and progressing clockwise.
Jump from boxes 1 to 4, landing as close to the exact middle of the box as possible.
Variations include reversing order from box 4 to 1 and adding diagonal patterns such as 1, 3, 4, and 2.
• Incorporate multidirectional sport-specific proprioceptive exercises once the athlete is able to perform the proprioceptive exercises described in the reparative phase.
-On level ground, begin single-leg standing exercises incorporating sport-specific exercises such as kicking a ball, catching and throwing a ball, or swinging a bat.
Progress to standing on an air-filled or foam cushion.
-Begin by keeping the exercise, such as catching a ball, close to the body.
Progress to throwing the ball farther from the body to force the athlete to shift his center of gravity.
• Ladder drills can be incorporated into the athlete’s program.
This stage is used to create exercises and movement patterns that will increasingly challenge the neuromuscular coordination of the injured athlete.
Example A—side shuffle: The athlete begins with both feet at position 1 where the L and R are located.
Move the left foot first to the outside of the box in front, then the right into the box next to it. Proceed as depicted.
Example B—carioca: Step over with the left leg first, then behind with the right leg.
The above exercises prepare the athlete to begin sport-specific drills.
The athlete must be able to demonstrate proficiency in stopping, changing direction, jumping, and landing as pertaining to the specific sport before returning to practice or competition.
A supportive ankle brace or taping has been shown to be an effective aid in preventing reinjury without impeding performance.
Technical training (on jumping and landing), proprioceptive training, and orthosis have all been shown to decrease the risk of reinjury, with technical training and proprioceptive training being more effective in those with a history of 4 or more sprains.
Poor compliance with therapy may predispose to functional instability of the ankle.
The athlete may complain of impeded sports performance due to the feeling that the ankle is unstable and fear of re-injury.
Although the injured ligament may have regained preinjury tensile strength, complete proprioception rehabilitation may take longer.
Upon initial return to sport, the athlete should continue to support the ankle with tape or a brace.
To prevent reinjury, proprioception rehabilitation should continue until the athlete demonstrates improved functional skills particular to the individual sport.
After being discharged from care, the athlete should be encouraged to continue a home exercise program to ensure full restoration of impairments and to prevent re-injury.
McHugh et al83 had football players with a history of ankle sprains perform single-leg stances on a foam stability pad for 5 minutes for each leg.
This was done 5 days a week for 4 weeks of preseason and 2 days a week for 9 weeks during the season.
This was the only preventative exercise used and was done on their own.
The study showed a 77% decrease in injury incidence.
A study by Hupperts et al84 showed a similar decrease in injury risk with unsupervised exercises, demonstrating that a home program can be an effective tool in rehabilitation.
Surgical treatment:
Syndesmotic screw fixation
Technique:
two 3.5 or 4.5 mm syndesmotic screws through 3 or 4 cortices placed 2-5 cm above the plafond
screw material
no difference between stainless steel and titanium screws
bioabsorbable screws with similar outcomes
number of cortices
no difference between 3 or 4 cortices
number of screws
fixation with two screws is preferable
position of the foot during fixation
a recent study challenges the principle of holding the ankle in maximal dorsiflexion to avoid overtightening
Postoperative:
typically non-weight-bearing for 6-12 weeks
may prolong if screw breakage is a concern
Physical Therapy Treatment
Goals :
First two weeks: ROM, decrease pain and swelling, protect ligaments against further injury
Week 3 and onward: Restore normal ROM, strengthen ligaments and supporting muscles, training to improve endurance and balance (Level of evidence 5)
The most important long-term goal is to prevent re-injury!(Level of evidence 1A)
Patient Education:
Surgeon/PT’s recommended weight-bearing protocol
Caution against vigorous physical activity until full weight bearing and dynamic balance have normalized. (Level of evidence 3A)
Gait training with crutches or boot/brace [2](Level of evidence 1A)
Fall risk
Assistive Devices
Crutches- must be maintained until normal, pain-free gait is obtained
Walking boot or stirrup brace for unstable injuries
Modalities:
RICE (rest, ice, compression, elevation) initially for 15 min 3x a day. (However, Bleakley et al suggested that there is little evidence to support the use of RICE, although it is a widely accepted treatment
Non-steroidal anti-inflammatory drugs and comfrey ointment have been shown to improve short-term recovery following acute ankle sprain.
Therapeutic Exercise/ Neuromuscular Re-education:
First two weeks:
AROM flexion, ankle alphabet, dorsiflexion/plantarflexion, and inversion/eversion with theraband
Weeks 3-4:
Standing Stretch, seated dorsiflexion stretch with theraband, double heel raise progressing to single heel raise, and dorsiflexion stretching on step stool
Progressive weight-bearing and treadmill gait training to promote normal gait patterns.
Neuromuscular Re-education:
ankle proprioception, postural reflexes, and balance
Exercise:
Single-leg stance, disk or balance pad training, aquatic therapy
Progress to jogging, cycling, agility, jumping, and sport-specific drills.(Level of evidence 3A)
Modify exercises to avoid hyperdorsiflexion (stresses mortise joint), subtalar eversion, and loaded external rotation.
Manual Therapy :
Passive accessory movement of the talocrural and subtalar joints and passive stretching may help stiffness.(Level of evidence 3A)
Green et al: those subjects who used RICE with manual therapy were more likely to reach this normal ROM within the first 2 weeks of the ankle sprain than those who received RICE alone.
Collins et al: Subjects showed immediate ROM gains when Mulligan’s movement with mobilization was applied in the sub-acute sprains and in patients with recurrent sprains.
Landrum et al: Reported that one 30-second A-P talocrural joint mob immediately increased ankle dorsiflexion ROM after prolonged mobilization.
Complications:
Posttraumatic tibiofibular synostosis
incidence:
~10% after Weber C ankle fractures
treatment:
surgical excision:
reserved for persistent pain that fails to respond to nonsurgical management
ossification must be “cold” on bone scintigraphy prior to removal
2 Comments